Evidence of the climate control of strong seismicity in the Italian Apennines through groundwater recharge
- National Institute of Oceanography and Applied Geophysics—OGS, Udine, Italy
This work explores the possibility that the destructive earthquakes occurring along the Apennine Chain in Italy are systematically triggered by groundwater recharge. The focus is on multi-year transitions toward phases of wet climate rather than on short-term heavy rainfall occurring in a few days or seasonally. The analysis takes into consideration the earthquakes with a moment magnitude of Mw ≥5.8 that have occurred since 1901. Their time distribution is compared with the fluctuations of the self-calibrated Palmer Drought Severity Index (scPDSI), an indicator of soil moisture, here assumed to be a proxy for groundwater recharge. It is found that the scPDSI evolved through six main oscillations lasting from 11 to 25 years, and that, with one exception, the strongest earthquake in each phase is placed within 2 years from the maximum of soil moisture. Based on a statistical test for pairs of point processes, such a coincidence indicates a significant synchrony between the two phenomena. In particular, the two strongest earthquakes of the study period (the 1915 Marsica earthquake and the 1980 Irpinia–Basilicata earthquake, with moment magnitudes of Mw 7.1 and 6.8, respectively) occurred exactly in the year of two of the largest peaks of scPDSI. The connection between wet climate conditions and the occurrence of strong earthquakes is further investigated by comparing the time distribution of Mw ≥6.1 historical earthquakes that have occurred since 1200 AD with the evolution of the Great Aletsch Glacier in Switzerland, representative of water accumulation at the continental level. Even in this case, the earthquakes clustered during time periods of increased precipitation and lower water evaporation, corresponding to the extreme phases of the Little Ice Age. The agreement of the results at different time scales and using different climate indexes leads to postulate a significant and systematic role of groundwater recharge in the triggering of large earthquakes along the Apennines. It is also suggested that the earthquakes might be triggered by pore-pressure propagation, where the necessary hydraulic continuity is made possible by the intersection of the shallow karst structures with the seismogenic faults.
1 Introduction
It has been largely demonstrated by observations and models that surface water accumulation and its diffusion in the crust can interact with plate tectonics and trigger earthquakes, mainly by activating faults that are near to their rupture conditions (Wang and Manga, 2010). Basically, the triggering is attributed to the combination of two mechanisms (Mulargia and Bizzarri, 2014): the increase of elastic stress due to the water load; the flow of water in depth and the consequent diffusion of pore pressure to faults. The effect can be enhanced under geological conditions that may favor water accumulation and its migration down into the crust of the Earth. This is the case of the karst environments, with their developed systems of fractures and cavities. For karstic areas, it has been shown that intense rainfall can trigger minor seismicity. As reported by Miller (2008), cases were observed at Mt. Hochstaufen in Germany for the extreme precipitation of March and August 2002 (Hainzl et al., 2006; Kraft et al., 2006); in the Gard region of southern France after a catastrophic storm in September 2002 (Rigo et al., 2008); and in two distinct regions of Switzerland in August 2005 (Husen et al., 2007). Differently from the studies mentioned previously, the main interest here is on the possible systematic triggering of strong earthquakes over large karstic areas, a theme originally addressed for the seismicity of Greece (Liritzis and Petropoulos, 1992, Liritzis and Petropoulos, 1994). In a previous statistical study conducted in the pre-Alpine karstic belt of northeastern Italy (Bragato, 2021a), it has been shown that the advent of multi-year wet periods (characterized by increased precipitation and reduced evaporation due to lower temperatures) is almost a necessary precondition for the occurrence of damaging and destructive earthquakes. The Apennines, with the exclusion of their southernmost part, are another zone of Italy where strong seismicity (moment magnitude up to 7.2) and carbonate rock outcrops coexist (Figure 1), suggesting a possible significant role of precipitation in earthquake triggering, like for the 1980 Irpinia Mw 6.8 earthquake (D’Agostino et al., 2018). This theme is reconsidered here from an observational point of view by analyzing long time series of strong seismicity and climate data. The study specializes in the Apennines and improves with the use of instrumental meteorological data a previous work concerning the entire Italy in the last millennium (Bragato and Holzhauser, 2019). The latter article stood as evidence for a strict time correlation between the Italian strong seismicity and the most severe phases of the Little Ice Age (LIA, in the following, occurred roughly between 1300 AD and 1850 AD), characterized by cold/wet climate conditions across Europe. In the following, the time distribution of Mw ≥5.8 earthquakes that have occurred along the Apennines since 1901 is compared with the trend of the self-calibrated Palmer Drought Severity Index (scPDSI, Wells et al., 2004), which is an indicator of soil moisture computed from measures of precipitation and temperature. Further analysis back to 1200 AD is performed using the length of the Great Aletsch Glacier (GA-length in the following) reconstructed by Holzhauser (1997) as a proxy for wet climate conditions along the Apennines. This is because the GA-length is a reliable climate indicator used in several climate studies in Europe and is representative of glacial and, more in general, water accumulation in West-Central Europe (Holzhauser et al., 2005). The connection with the climate trend in the Apennines is supported by historical accounts, especially for the LIA (Grove, 2001). The results of the analysis are summarized and discussed at the end of the article, with some considerations on the physical mechanism by which groundwater recharge could trigger earthquakes in the study area.
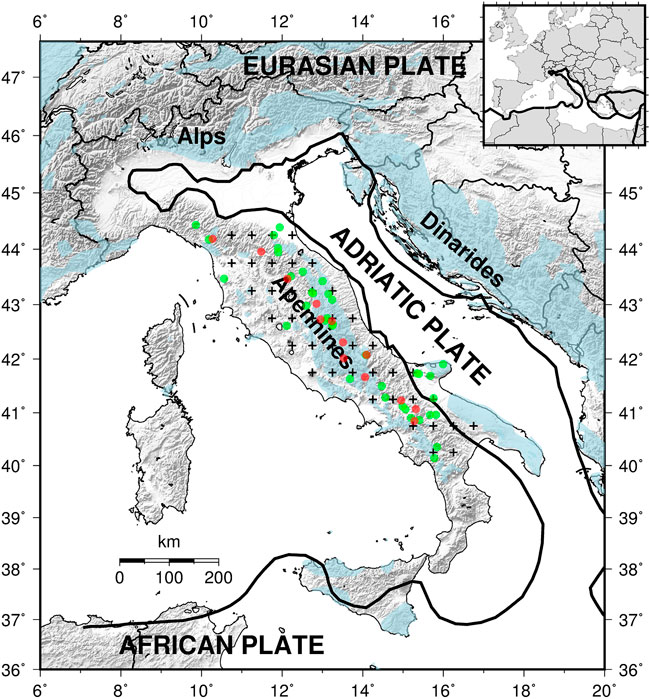
FIGURE 1. Tectonic setting of Italy with the indication of the epicenters of the earthquakes used in this work: in green, Mw ≥6.1 earthquakes occurred between 1200 and 1900; in red, Mw ≥5.8 earthquakes occurred since 1901. The small crosses are the grid points of the scPDSI dataset used to estimate the average scPDSI time series in Figure 2. In light blue are the carbonate rock outcrops (https://www.fos.auckland.ac.nz/our_research/karst/).
2 Data
The author considered the mainshocks extracted from the Italian seismic catalog CPTI15, version 2.0 (Rovida et al., 2019, 2020) with a moment magnitude of Mw ≥5.8, occurred inland in Italy since 1901 and have epicenters located between latitude 40.0°N and 44.5°N (red points in Figure 1). The selected area [see Fracassi and Valensise, (2007) for an overview of its geological and seismotectonic characteristics] comprises the portion of the Apennines characterized by significant outcrops of carbonate rock (light blue shadow in Figure 1), excluding the southernmost part of the chain in the Calabrian Arc. After removing the aftershocks using the declustering algorithm by Gardner and Knopoff, (1974), the selected dataset comprises the 13 earthquakes described in Table 1, whose magnitude and time distribution are represented by the red bars in Figure 2. The earthquakes produced macroseismic effects ranging from heavy damage and collapse of a few buildings up to generalized destruction (maximum macroseismic intensity between VIII and XI in the Mercalli–Cancani–Sieberg scale), in all the cases with victims (Guidoboni et al., 2018, 2019). The two strongest and most lethal earthquakes were those of 1915 (Marsica earthquake, Mw 7.1) and 1980 (Irpinia–Basilicata earthquake, Mw 6.8). with 32,610 and 2,735 casualties, respectively. Another highly destructive earthquake occurred in 1930 (Irpinia earthquake, Mw 6.7, 1,404 casualties). The present work focalizes on high-magnitude earthquakes (Mw≥5.8) because they have a significant social impact and are well representative of the seismic hazard of the country. There are also practical advantages on selecting a high-magnitude threshold: in general, the earthquakes are well documented; furthermore, they are more separated in space and time, so that the declustering of the seismic catalog is less critical.
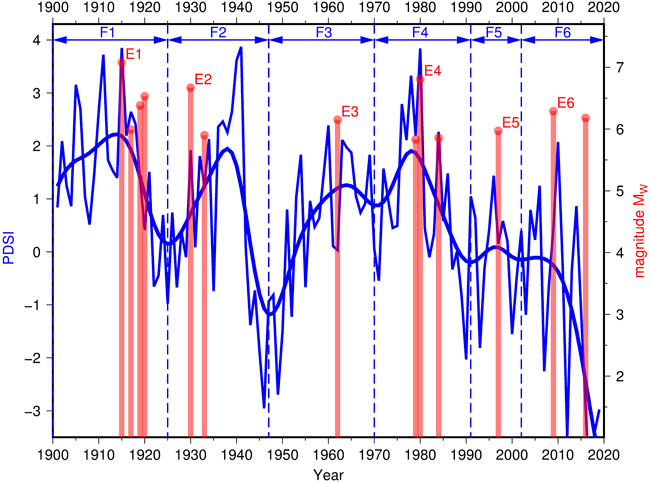
FIGURE 2. Relationship between the occurrence of M ≥5.8 earthquakes (red bars with the height proportional to the magnitude) and the evolution of the scPDSI (thin blue line) in the Apennines since 1901. The thick blue line is the smoothed version of the scPDSI obtained by kernel regression for a smoothing parameter h=3 years. The time periods F1–F6 are those corresponding to the six long-term fluctuations of the smoothed scPDSI. The earthquakes E1–E6 are the strongest earthquakes in each of the time periods F1–F6.
The climate trend in the Apennines since 1901 is summarized here by the self-calibrated Palmer Drought Severity Index (scPDSI; Wells et al., 2004), an evolution of the original PDSI by Palmer (1965), which is computed combining instrumental measures of precipitation and temperature with a simplified model of water absorption and evaporation in an agricultural soil. Its values range between −10 for extremely dry conditions to 10 for extremely wet conditions. The author used the time series available from the Climatic Research Unit of the University of East Anglia (van der Schrier et al., 2013; Barichivich et al., 2021). The values here considered are those averaged over the grid of points corresponding to the study area (black crosses in Figure 1) for the summer months of June, July, and August (thin blue line in Figure 2). The summer-averaged scPDSI is almost a standard in climate studies related to agriculture; this is because it represents the soil moisture during the main period of growth and maturation of crops, having a direct effect on their final outcome. It is offered as the default value by some data repositories, like the Old-World Drought Atlas (OWDA, Cook et al., 2015). Its adoption allows a direct comparison with other investigations on the long-term climate trends (e.g., van der Schrier et al., 2006). The scPDSI is devised specifically for agricultural soils and not for the infiltration of water into the crust. Nonetheless, in many areas, it correlates well with the water storage changes estimated from GRACE (Gravity Recovery and Climate Experiment) satellites (Dai, 2011). It has been used successfully as an indicator of groundwater recharge in comparison with the occurrence of strong earthquakes in northeastern Italy (Bragato, 2021a) and Southern California (Bragato, 2021b).
For the study of the time period between 1200 AD and 1900 AD, the author used the mainshocks with a moment magnitude of Mw ≥6.1 drawn from the seismic catalog CPTI15 as mentioned previously (green circles in Figure 1, a total of 28 earthquakes occurred before 1900) in comparison with the GA-length reconstructed by Holzhauser (1997). Concerning the choice of the magnitude threshold for earthquakes, ideally one should adopt the magnitude threshold that guarantees the completeness of the catalog throughout the entire study period. The analysis performed by Stucchi et al. (2011) is extremely conservative and, for magnitude Mw ≥6.1 in the entire study area, gives completeness starting from 1530 AD. An alternative approach admits that the earthquake catalog is incomplete and looks at its quality (i.e., the probability that earthquakes over a given magnitude threshold are reported in the catalog). Bragato (2018) showed that the quality of the Italian catalog between 1400 and 1550 AD is not worse than that in the time period 1650–1850 AD. This result is supported also by historical considerations and a formal statistical comparison with a reconstruction of the gross domestic product (GDP) per capita of Italy since 1310. In particular, the time period 1400–1550 corresponds to the Renaissance, an epoch characterized by significant economic and cultural growth, more favorable for the reporting of earthquake news in the written form. In the present work, by choosing the magnitude threshold Mw ≥6.1, the author assumes that the quality of the catalog is almost homogeneous, at least since 1400. This means that, even if not all the earthquakes with Mw ≥6.1 are reported, the relative proportion of earthquakes through time should be preserved. Differently, the time density of the earthquakes that occurred before 1400 should be carefully considered, taking into account that the quality of the catalog might be low.
3 Analysis
The origin time of the strongest earthquakes that hit the Apennines since 1901 and the time series of the scPDSI are matched in Figure 2 (vertical red bars and thin blue line, respectively). In order to emphasize the multi-year climate oscillations, in Figure 2 it is also traced the smoothed version of the scPDSI estimated by kernel regression (Bowman and Azzalini, 1997) using the smoothing parameter h = 3 years (thick blue line). The smoothed scPDSI has six fluctuations covering the time periods F1–F6, as shown in Figure 2. The first four of them have large amplitude and a similar time duration (21–24 years). This behavior changes after 1990. The last two smoothed oscillations have small amplitude compared to the corresponding yearly peaks (thin line with local maxima in 1996 and 2010, respectively): it means that the transition from dry to wet conditions was more rapid than in the past (e.g., three years from the minimum of 2007 to the maximum of 2010). Independent on the duration and the amplitude of the multi-year fluctuations, each of them is associated with at least one large earthquake. The labels E1–E6 in Figure 2 denote the strongest earthquake in each of the six climate phases. In five cases out of six, the strongest earthquake occurred within 2 years of the yearly peak of the scPDSI. The only exception is the earthquake of 1930 (E2 in Figure 2): it occurred in a year of a large increase in scPDSI (from −0.1 in 1929 to 1.9 in 1930) but anticipated by 11 years the peak of 1941. The earthquake E6 (the L’Aquila earthquake of 6 April 2009, Mw 6.3) preceded the peak of scPDSI by 1 year but within a period of sustained precipitation and rapid increase of soil moisture after the minimum of 2007. It is also to be noted that the scPDSI has a long-term memory [9–18 months according to Vicente-Serrano et al. (2010)] with consequent inertia, so that it reacts with delay to the changes in the precipitation regime. The author checked if the proportion of five earthquakes out of six within 2 years from the peak of scPDSI represents a statistically significant synchrony between the two phenomena. For this purpose, the author used the statistical test by Doss (1989), implemented in the K1D software package (Gavin et al., 2006), and briefly described for a similar application in Bragato (2021a). In general, based on two series of event times and the overall observation time (120 years for the present case), it estimates the statistics
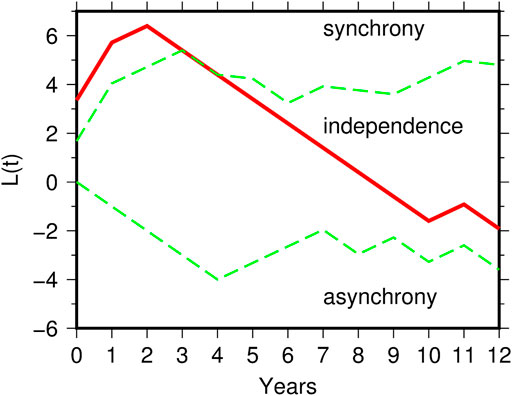
FIGURE 3. Test for synchronization between the earthquakes E1–E6 in Figure 2 and the peak of the scPDSI in each of the fluctuations F1–F6. The three curves indicate the statistic L(t) (continuous line) and its 95% confidence envelope (dashed lines).
Figure 4 illustrates the relationship between the mainshocks with Mw ≥6.1 and the evolution of the GA-length in the time period between 1200 AD and 1900 AD. The GA-length has three large fluctuations, corresponding to the extreme phases of the LIA. The earthquakes are clustered in the earliest period of climate deterioration between 1250 and 1350 AD and, cumulatively, around the two glacial pulses from 1600 to 1850. The earthquakes were extremely rare during the period of mild climate between 1350 and 1600, although in this time period the area was struck by the strongest known earthquake [Sannio–Molise earthquake of 1456, Mw 7.2 (Fracassi and Valensise, 2007)]. The strong seismic activity temporarily suspended after the peak of the last glacial episode in the 19th century (the last event was the 1857 Basilicata earthquake, Mw 7.1) and restarted 58 years later with the 1915 Marsica earthquake, corresponding to the earliest large peak of scPDSI (Figure 2). As discussed in the previous Data section, there is the possibility that the time distribution of the historical earthquakes is distorted by the variations in the completeness and quality of the catalog through time. Based on completeness analysis performed by Stucchi et al. (2011), both the low seismic activity after 1530 AD and the sudden acceleration that occurred one century later should be reliable features. According to Bragato (2018), the quality of the catalog between 1400 and 1530 AD is not worse than that in the following period, so that the relative scarcity of earthquakes should reflect that of the true (partially unknown) seismic activity. Alternatively, following Stucchi et al. (2011), the completeness back to 1400 AD is guaranteed by restricting the earthquakes to Mw ≥6.6 (red bars in Figure 4): even in this case, the great divergence pre/post 1600 AD is confirmed. Completeness and quality analyses give no useful indication for the time period 1200–1400 AD. Nonetheless, based on historical considerations, it appears reasonable to assume that the quality of the catalog after 1400 AD is not worse than that in the previous period (i.e., the proportion of “lost” earthquakes does not increase after 1400 AD), and that the time distribution of earthquakes in Figure 4 depicts a real deceleration of activity after a peak around 1300 AD.
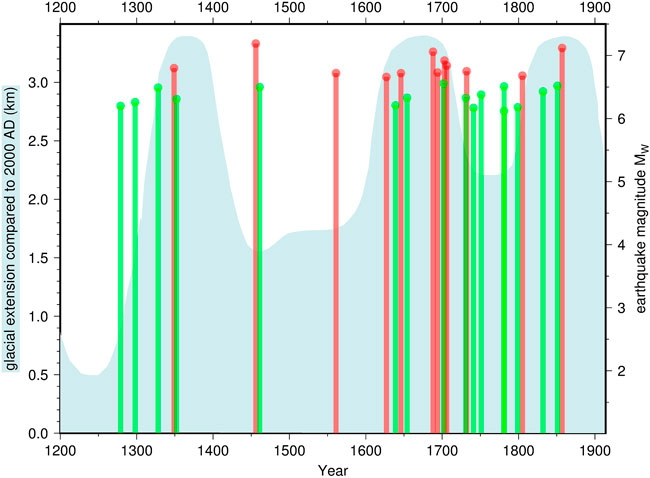
FIGURE 4. Relationship between the occurrence of Mw ≥6.1 earthquakes along the Apennines (bars with the height proportional to the magnitude, those with Mw ≥6.6 in red and those with Mw <6.6 in green) and the extension of the Great Aletsch Glacier between 1200 and 1900 AD (light blue area).
4 Discussion and conclusion
The comparison between the occurrence of strong earthquakes and the trend of soil moisture in the Apennines since 1901 indicates a similar time modulation, with the strong earthquakes that clustered near to the maxima scPDSI in five of the six climate fluctuations occurred so far. Based on the statistical test for synchrony, the time relationship is recognized above the 95% confidence level. In particular, the two strongest earthquakes in the catalog took place in the same year of the corresponding peak of the scPDSI (the 1915 Marsica earthquake, Mw 7.1, and the 1980 Irpinia–Basilicata earthquake, Mw 6.8). In a complementary way, the lack of seismicity characterizes the dry periods, especially those centered on 1925, 1947, and 1991 AD. Analyzing the strong seismicity back to 1200 AD, it is shown that, with a few exceptions, the main earthquakes clustered around the glacial peaks of the LIA, when also the climate in the peninsular Italy turned to cold/wet conditions. Even in the historical case, a very low seismic rate, like that occurred between 1400 and 1600 AD, is associated with mild climate conditions.
Under the hypothesis that scPDSI and glacial expansion are representative of the groundwater recharge in the Apennines, the results outlined so far suggest a stable connection with the occurrence of earthquakes. Based on a large body of similar evidence collected worldwide as well as on geophysical models, it is possible to postulate a triggering effect by water accumulation (Wang and Manga, 2010). The novelty emerged here is that the relationship is not episodic but systematic and persistent in the Apennines, at least in the last 800 years, involving the largest part of seismicity. This behavior resembles that observed for similar karst conditions in northeastern Italy (Bragato, 2021a) and relates the earthquake occurrence to multi-year climate fluctuations rather than to short-term meteorological effects. From the climatic point of view, the curves of scPDSI in Figure 2 indicate two change points: the first one around 1990, with the suppression of the 20-year large oscillations; the second one after 2010, marked by the turn toward the driest climate conditions of the last 120 years, with the minima of the scPDSI reached in 2012, 2017, 2018, and 2019. These variations have repercussions on the relationship with the strong earthquakes that, since 1997, are associated to accelerations of scPDSI lasting only a few years.
The author has mentioned in the Introduction that the triggering effect of water is commonly explained by two mechanisms: the instantaneous increase in elastic stress on faults as a consequence of the water load; the pore pressure diffusion thanks to the hydraulic continuity from the surface to the seismogenic layer. These mechanisms are discussed by D’Agostino et al. (2018) for the specific case of the Irpinia Fault Zone (Southern Apennines). By analyzing the trend of minor seismicity in the time period 2008–2017, they observe a delay of a few months with respect to the seasonal hydraulic loading, so that they exclude the instantaneous intervention of the elastic stress. On the other hand, the hydraulic continuity required by the pore pressure propagation seems to be incompatible with the geological setting of the area. Following Segall (1989), they suggest an alternative model comprising two layers separated by a low-permeability barrier: the upper karstic layer absorbs water and deforms horizontally, driving a similar deformation in the lower layer by elastic coupling. It is hypothesized that this mechanism could have triggered the 1980 Irpinia–Basilicata earthquake under the combined stimulus of the multi-year hydraulic load (the same depicted in Figure 2 for the scPDSI) and the heavy rainfall that preceded the earthquake by about 40 days. In this model, the adjunctive elastic stress is of the order of a few kPa/year, which is at the limit for a triggering effect. Another possibility, not explored in the cited article, is that the hydraulic continuity exists and is achieved by the intersection of the karstic layer with the most superficial part of the fault system. It is speculated here that under this condition, the deep portion of the faults nucleating strong earthquakes (around 10 km deep) could be affected by a considerable portion of the high pressure that develops in the karstic conduits due to the water recharge [e.g., oscillation of the order of 300 kPa for the hydraulic cycle 2015–2016 measured under the Gran Sasso Massif in Central Italy (De Luca et al., 2018)].
Data availability statement
Publicly available datasets were analyzed in this study. These data can be found at: Italian seismic catalog at https://doi.org/10.13127/CPTI/CPTI15.2; time series of scPDSI at https://crudata.uea.ac.uk/cru/data/drought/.
Author contributions
The author confirms being the sole contributor of this work and has approved it for publication.
Funding
This study was carried out with contributions from the program POR-FESR Regione Veneto 2014–2020, Action 5.3.1.
Acknowledgments
All the figures were produced using the Generic Mapping Tools (Wessel et al., 2019).
Conflict of interest
The author declares that the research was conducted in the absence of any commercial or financial relationships that could be construed as a potential conflict of interest.
Publisher’s note
All claims expressed in this article are solely those of the authors and do not necessarily represent those of their affiliated organizations, or those of the publisher, the editors, and the reviewers. Any product that may be evaluated in this article, or claim that may be made by its manufacturer, is not guaranteed or endorsed by the publisher.
References
Barichivich, J., Osborn, T. J., Harris, I., van der Schrier, G., and Jones, P. D. (2021). “Monitoring global drought using the self-calibrating palmer drought severity index,” in State of the climate in 2020 (Bulletin of the American Meteorological Society 102). Editors R. J. H. Dunn, F. Aldred, N. Gobron, J. B. Miller, and K. M. Willett, S68–S70. doi:10.1175/BAMS-D-21-0098.1
Bowman, A. W., and Azzalini, A. (1997). Applied smoothing techniques for data analysis. Oxford, United Kingdom: Oxford University Press.
Bragato, P. L., and Holzhauser, H. (2019). Observations on the connection between glacial phases, natural catastrophes and economic trends of the last millennium in Italy. Holocene 29, 1322–1334. doi:10.1177/0959683619846984
Bragato, P. L. (2018). Past economic conditions affect the reconstruction of the seismic history of Italy. Seism. Res. Lett. 89, 2404–2410. doi:10.1785/0220180129
Bragato, P. L. (2021a). Systematic triggering of large earthquakes by karst water recharge: Statistical evidence in northeastern Italy. Front. Earth Sci. (Lausanne). 9, 664932. doi:10.3389/feart.2021.664932
Bragato, P. L. (2021b). Statistical relationship between the decrease of major seismicity and drought in southern California since 1900. Front. Earth Sci. (Lausanne). 9, 790412. doi:10.3389/feart.2021.790412
Cook, E. R., Seager, R., Kushnir, Y., Briffa, K. R., Büntgen, U., Frank, D., et al. (2015). Old world megadroughts and pluvials during the common era. Sci. Adv. 1, e1500561. doi:10.1126/sciadv.1500561
D’Agostino, N., Silverii, F., Amoroso, O., Convertito, V., Fiorillo, F., Ventafridda, G., et al. (2018). Crustal deformation and seismicity modulated by groundwater recharge of karst aquifers. Geophys. Res. Lett. 45, 12253–12262. doi:10.1029/2018GL079794
Dai, A. (2011). Characteristics and trends in various forms of the palmer drought severity index during 1900–2008. J. Geophys. Res. 116, D12115. doi:10.1029/2010JD015541
De Luca, G., Di Carlo, G., and Tallini, M. (2018). A record of changes in the Gran Sasso groundwater before, during and after the 2016 Amatrice earthquake, central Italy. Sci. Rep. 8, 15982. doi:10.1038/s41598-018-34444-1
Doss, H. (1989). On estimating the dependence between two point processes. Ann. Stat. 17, 749–763. doi:10.1214/aos/1176347140
Fracassi, U., and Valensise, G. (2007). Unveiling the sources of the catastrophic 1456 multiple earthquake: Hints to an unexplored tectonic mechanism in southern Italy. Bull. Seismol. Soc. Am. 97, 725–748. doi:10.1785/0120050250
Gardner, J. K., and Knopoff, L. (1974). Is the sequence of earthquakes in southern California, with aftershocks removed, Poissonian? Bull. Seismol. Soc. Am. 64, 1363–1367. doi:10.1785/bssa0640051363
Gavin, D. G., Hu, F. S., Lertzman, K., and Corbett, P. (2006). Weak climatic control of stand-scale fire history during the late Holocene in southeastern British Columbia. Ecology 87, 1722–1732. doi:10.1890/0012-9658(2006)87[1722:wccosf]2.0.co;2
Grove, A. T. (2001). The “Little Ice Age” and its geomorphological consequences in mediterranean Europe. Clim. Change 48, 121–136. doi:10.1023/A:1005610804390
Guidoboni, E., Ferrari, G., Mariotti, D., Comastri, A., Tarabusi, G., Sgattoni, G., et al. (2018). CFTI5Med, catalogue of strong earthquakes in Italy (461 B.C.-1997) and mediterranean area (760 B.C.-1500). Rome, Italy: Istituto Nazionale di Geofisica e Vulcanologia INGV. doi:10.6092/ingv.it-cfti5
Guidoboni, E., Ferrari, G., Tarabusi, G., Sgattoni, G., Comastri, A., Mariotti, D., et al. (2019). CFTI5Med, the new release of the catalogue of strong earthquakes in Italy and in the Mediterranean area. Sci. Data 6, 80. doi:10.1038/s41597-019-0091-9
Hainzl, S., Kraft, T., Wassermann, J., Igel, H., and Schmedes, E. (2006). Evidence for rainfall-triggered earthquake activity. Geophys. Res. Lett. 33, L19303. doi:10.1029/2006GL027642
Holzhauser, H. (1997). “Fluctuations of the grosser Aletsch Glacier and the gorner glacier during the last 3200 Years – new results,” in Glacier fluctuations during the holocene (Paläoklimaforschung/Palaeäoclimate research 24). Editor B. Frenzel (Stutgart, Germany: Gustav Fischer Verlag). 35.
Holzhauser, H., Magny, M., and Zumbühl, H. Z. (2005). Glacier and lake-level variations in west-central Europe over the last 3500 years. Holocene 15, 789–801. doi:10.1191/0959683605hl853ra
Husen, S., Bachmann, C., and Giardini, D. (2007). Locally triggered seismicity in the central Swiss Alps following the large rainfall event of August 2005. Geophys. J. Int. 171, 1126–1134. doi:10.1111/j.1365-246x.2007.03561.x
Kraft, T., Wassermann, J., Schmedes, E., and Igel, H. (2006). Meteorological triggering of earthquake swarms at Mt. Hochstaufen, SE-Germany. Tectonophysics 424, 245–258. doi:10.1016/j.tecto.2006.03.044
Liritzis, I., and Petropoulos, B. (1994). The relationship between precipitation and shallow earthquakes (M≥5 1/2) revisited. Eur. Seism. Comm. XXIV. Gen. Assem. 1, 1973.
Liritzis, Y., and Petropoulos, B. (1992). A preliminary study of the relationship between large earthquakes and precipitation for the region of Athens, Greece. Earth Moon Planets 57, 13–21. doi:10.1007/bf00115412
Miller, S. A. (2008). Note on rain-triggered earthquakes and their dependence on karst geology. Geophys. J. Int. 173, 334–338. doi:10.1111/j.1365-246X.2008.03735.x
Mulargia, F., and Bizzarri, A. (2014). Anthropogenic triggering of large earthquakes. Sci. Rep. 4, 6100. doi:10.1038/srep06100
Palmer, W. C. (1965). Meteorological drought. Res. Paper No.45. Washington D.C, USA: Dept. of Commerce.
Rigo, A., Béthoux, N., Masson, F., and Ritz, J.-F. (2008). Seismicity rate and wave-velocity variations as consequences of rainfall: The case of the catastrophic storm of september 2002 in the nîmes fault region (gard, France). Geophys. J. Int. 173, 473–482. doi:10.1111/j.1365-246X.2008.03718.x
Rovida, A., Locati, M., Camassi, R., Lolli, B., and Gasperini, P. (2019). Catalogo Parametrico dei Terremoti Italiani (CPTI15), versione 2.0. Rome, Italy: Istituto Nazionale di Geofisica e Vulcanologia INGV. doi:10.13127/CPTI/CPTI15.2
Rovida, A., Locati, M., Camassi, R., Lolli, B., and Gasperini, P. (2020). The Italian earthquake catalogue CPTI15. Bull. Earthq. Eng. 18, 2953–2984. doi:10.1007/s10518-020-00818-y
Segall, P. (1989). Earthquakes triggered by fluid extraction. Geol. 17, 942–946. doi:10.1130/0091-7613(1989)017<0942:ETBFE>2.3.CO;2
Stucchi, M., Meletti, C., Montaldo, V., Crowley, H., Calvi, G. M., and Boschi, E. (2011). Seismic hazard assessment (2003–2009) for the Italian building code. Bull. Seismol. Soc. Am. 101, 1885–1911. doi:10.1785/0120100130
van der Schrier, G., Barichivich, J., Briffa, K. R., and Jones, P. D. (2013). A scPDSI-based global data set of dry and wet spells for 1901-2009. J. Geophys. Res. Atmos. 118, 4025–4048. doi:10.1002/jgrd.50355
van der Schrier, G., Briffa, K. R., Jones, P. D., and Osborn, T. J. (2006). Summer moisture variability across Europe. J. Clim. 19, 2818–2834. doi:10.1175/JCLI3734.1
Vicente-Serrano, S. M., Beguería, S., López-Moreno, J. I., Angulo, M., and El Kenawy, A. (2010). A new global 0.5° gridded dataset (1901-2006) of a multiscalar drought index: Comparison with current drought index datasets based on the palmer drought severity Index. J. Hydrometeorol. 11, 1033–1043. doi:10.1175/2010JHM1224.1
Wang, C-Y., and Manga, M. (2010). Earthquakes and water. Berlin and Heidelberg, Germany: Springer-Verlag.
Wells, N., Goddard, S., and Hayes, M. J. (2004). A self-calibrating palmer drought severity index. J. Clim. 17, 2335–2351. doi:10.1175/1520-0442(2004)017<2335:ASPDSI>2.0.CO;2
Keywords: Apennines, climate change, precipitation, poroelastic effects, earthquake triggering
Citation: Bragato PL (2022) Evidence of the climate control of strong seismicity in the Italian Apennines through groundwater recharge. Front. Earth Sci. 10:1028152. doi: 10.3389/feart.2022.1028152
Received: 25 August 2022; Accepted: 12 September 2022;
Published: 05 October 2022.
Edited by:
Matteo Picozzi, University of Naples Federico II, ItalyReviewed by:
Yawar Hussain, University of Liège, BelgiumTony Alfredo Stabile, Consiglio Nazionale delle Ricerche, Istituto di Metodologie per l'Analisi Ambientale, Italy
Copyright © 2022 Bragato. This is an open-access article distributed under the terms of the Creative Commons Attribution License (CC BY). The use, distribution or reproduction in other forums is permitted, provided the original author(s) and the copyright owner(s) are credited and that the original publication in this journal is cited, in accordance with accepted academic practice. No use, distribution or reproduction is permitted which does not comply with these terms.
*Correspondence: Pier Luigi Bragato, pbragato@ogs.it