There is no shortage of superlatives that can be applied to the Large Hadron Collider near Geneva, though many are strange and unusual. For a start, the huge underground device, which batters beams of protons into each other at colossal energies, can fairly claim to be the coolest place on Earth. Bending protons as they hurtle round the LHC’s circular 27km tunnel turns out to be a chilly business.
Thousands of huge magnets are needed to control the beams and these have to work with complete efficiency. To achieve this, the device is refrigerated to two degrees above absolute zero on the thermodynamic temperature scale: -271C, a temperature at which electric currents flow without resistance. In this way, the collider’s magnets can work to their maximum potential.
“There is no place in the natural world that is anywhere as cold as the LHC,” says Ray Veness, a senior engineer at Cern, the European Organisation for Nuclear Research. “And given that we also do great science here, it is clear this has to be the coolest place on Earth.”
Surprisingly, the collider – which scientists used to discover the Higgs boson in 2013 – is also one of the hottest spots on Earth on occasions. When those beams of protons slam into each other, they create tiny explosions that mimic events just after the big bang birth of the universe and release intense bursts of energy. Some of these produce temperatures that are 100,000 times hotter than those at the sun’s core – albeit for only a fraction of a second and over a minuscule region of space.
And finally there is the vacuum inside the collider. This is the cleanest ever created by engineers. In order to prevent even the tiniest entity impeding those beams of protons as they whiz at near light speed around the collider, virtually every atom or molecule has been sucked from its tubes, creating a vacuum that is purer than that found in near-Earth space, millions of miles above our heads.
Thus Cern has constructed a device that is the world’s coolest, occasionally its hottest and whose contents are emptier than the vacuum of interplanetary space. It is a remarkable achievement. Yet it is simply not good enough, say the centre’s scientists and engineers. They want more – and are now completing an upgrade that will push the LHC to operate at even greater intensities and extremes. “The first run of the LHC – between 2008 and 2012 when we found the Higgs – was the collider’s maiden voyage,” says Veness. “Since then it has been in for a refit and now it is ready to show the world what it can really do.”
During its first run, protons were fired around the LHC at energies of up to 4 trillion electron volts (4 TeV). By making them crash head-on, this released bursts of energy of up to 8 TeV. From the resulting subatomic debris, scientists were able to pinpoint the existence of the Higgs boson. Now, Cern is finishing an upgrade that will allow those beams to run at 6.5 TeV and create collisions that will release up to 13 TeV. As Cern’s director for accelerators and technology, Frédérick Bordry, puts it: “The LHC is almost like a new machine now.”
The point is backed by Birmingham University’s Professor Dave Charlton, spokesman for the Atlas experiment, one of the major detectors fitted to the LHC. “With this increase in energy we will be able to create many more massive particles than we could three years ago.
“We will be moving into unexplored territory. Certainly we have far less of an idea what we might find compared with our first run, when we knew there was a good chance we would find the Higgs. This time we expect to uncover new physics, though we do have some goals. For a start, one of our main tasks will be to try to prove if a hypothesis called supersymmetry is true or not.”
Supersymmetry predicts that versions of the particles that make up normal matter possess mirror or supersymmetrical versions. Thus there could be supersymmetrical quarks – or squarks – out there. Or supersymmetrical electrons – selectrons. “The trouble is that supersymmetry predicts that these entities exist but doesn’t say at what energies we might find them,” adds Charlton.
Nevertheless, Cern physicists believe supersymmetrical particles could lie within the range of the energetic collisions that will be generated in the upgraded LHC. And if they do detect these strange entities, they could provide the solution to one of the universe’s greatest mysteries: the nature of dark matter.
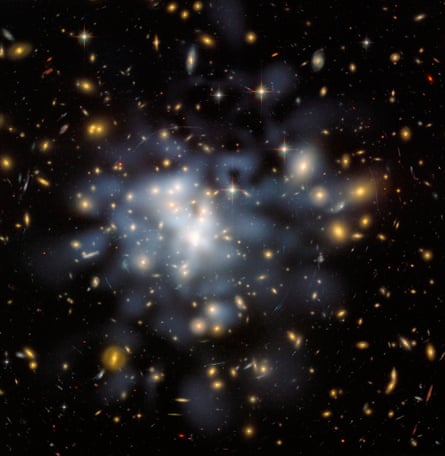
Dark matter is believed to pervade the cosmos, giving galaxies far greater masses than could be supplied by the “normal” matter of protons, neutrons and electrons. Its existence is only inferred, however, for despite decades of effort, scientists have yet to observe a single unit of dark matter. The new LHC could change that.
“The main candidate to explain dark matter is the Wimp – which stands for weakly interacting massive particle,” adds Charlton. “And it may turn out that Wimps are types of supersymmetrical particles that we will be able to make in the LHC. We will see lots and lots of events in our detectors, of course, and will have to disentangle what is going on. However, if we see collisions with missing energy in them – a sign that we might have created a supersymmetrical or dark matter particle that has carried away that energy – then that would suggest we are on to something.”
The prospects of finding supersymmetrical particles in the LHC’s colliders in the next two or three years is also rated as strong by leading Cern theorist Professor John Ellis, of King’s College London. “I favour the idea that one of the lighter supersymmetrical particles is one that accounts for dark matter. So the chances of dark matter appearing in the LHC’s detectors over the next couple of years look good, though it won’t appear directly. By its very nature, dark matter hardly interacts with normal matter and so will not appear in our detectors. However, it will be noticeable as an event that has missing energy and momentum. In that way, we will know we have created dark matter.”
The huge device is designed to satisfy many different scientific needs, including those of Professor Guy Wilkinson and his colleagues, whose LHCb spectrometer is located in the LHC tunnel. His team’s prime concern is the beauty quark, a particle whose behaviour is considered central to understanding another major puzzle about our cosmos: why is it dominated by matter and not antimatter?
Equal amounts of matter and antimatter were created at the big bang but the latter is now noticeable for its absence, despite astronomers’ efforts to detect it. This domination of matter over antimatter has come about by a process known as CP violation, scientists now believe, though this is still inadequately understood.
“We want to put that right,” adds Wilkinson. “The crucial point is that beauty quark and its antimatter equivalent break down in a particularly varied number of ways when you have created them in a collider. These different decay avenues will allow us to study in detail how one type of normal matter behaves in contrast to its antimatter corollary. Hopefully that will help us pinpoint the exact mechanism by which our universe has become dominated by matter and not antimatter.”
In short, a great deal is expected from the LHC once it has completed the big shutdown, as Veness describes the two-year refit. “It has taken between 1-2 million hours of work to get the machine ready,” he says. “With the LHC, you are always pushing the technology to the limit but we believe we have got there and should begin firing beams in early March. We should then have the collider running at full strength later in the year. And then the fun begins.”
Creating new particles
The Large Hadron Collider accelerates bunches of protons, in two beams, each less than a hair’s breadth in diameter, to near light speed. One beam circulates clockwise, the other anticlockwise. Chambers along the LHC use radio waves to pass energy to the protons as they hurtle past, making them move faster and faster.
Then, at four points along the LHC tunnel, the bunches of protons – each containing 100bn particles – are made to cross and collide. The huge energies released are converted into new matter and barrages of subatomic detritus are flung in all directions. These are then studied by the instruments in sets of detectors - Alice, Atlas, CMS and LHCb - that have been built around one of the four proton beam collision points.
In this way, scientists pinpointed the Higgs boson in 2013, a particle predicted 60 years earlier by Edinburgh University’s Peter Higgs and whose existence accounts for the mass of other subatomic particles. Now, the LHC has been upgraded to create even more energetic collisions and so generate even more massive particles.
“Protons are made up of particles called quarks which are held together by other subatomic entities called gluons,” says Charlton. “As far as we know, quarks and gluons are point-like entities - so when they collide they will annihilate each other totally and produce something new that could have all the energy of that collision.” Thus particles with the mass of several TeV could emerge from the upgraded LHC. The Higgs boson has a mass of around 125 GeV (the equivalent of 0.125 TeV).
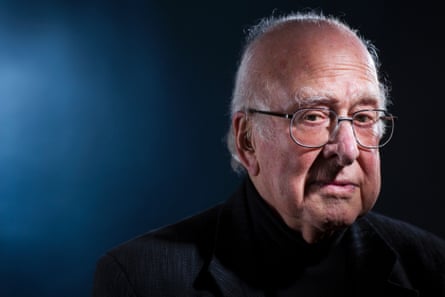
The search for dark matter
Astronomers discovered in the 1970s that distant galaxies were spinning too fast for their apparent mass. At the rotation speeds that were observed, these galaxies should have been flying apart. They possessed insufficient normal matter – in the form of protons, neutrons and molecules – to account for their rotation speeds. Only the existence of extra unseen mass – dubbed dark matter – could explain the observation. But what could dark matter be made of?
Many candidates were proposed. For example, it was suggested that failed stars called brown dwarves might pepper the void between stars and give galaxies extra mass and gravity. But these failed to materialise, leaving scientists with only one real candidate: Wimps, or weakly interacting massive particles. However, as their names suggest, these particles interact only very weakly with particles that make up standard matter. Hence the problem detecting them.
At present, researchers are trying to pinpoint dark-matter Wimps in two ways. The first has involved building detectors deep below ground. Down there, all other particles, including those generated by cosmic rays that bombard Earth’s surface, are blocked out. When a Wimp makes a rare interaction with normal matter it should then reveal itself in detectors. But so far this approach has had no success.
The second method is to try to make dark matter – in the form of supersymmetrical particles – by generating them in the LHC. As Charlton says: “Supersymmetry particles provide a perfectly natural explanation for dark matter.”
Comments (…)
Sign in or create your Guardian account to join the discussion